Solar radiometer vs pyranometer
A solar radiometer is a device that reacts to the power of sunlight. The Crookes radiometer was the first of its kind. Nowadays, we can also build more sophisticated solar radiometers that not only react to the power of sunlight but also measure it. They are called pyranometers. This article explains the history of the Crookes radiometer, how it works, and what the differences are compared to a pyranometer.
Crookes radiometer
In 1873, the chemist Sir William Crookes invented a solar radiometer (or light-mill). He did this while investigating the weights of samples in a partial vacuum chamber when he noticed something interesting. If the sample was under sunlight, the weight measurement became inconsistent. This led him to inspect this behaviour further and create the Crookes radiometer as we know it today.
What does a solar radiometer look like?
Figure 1 shows a schematic of a typical Crookes solar radiometer.
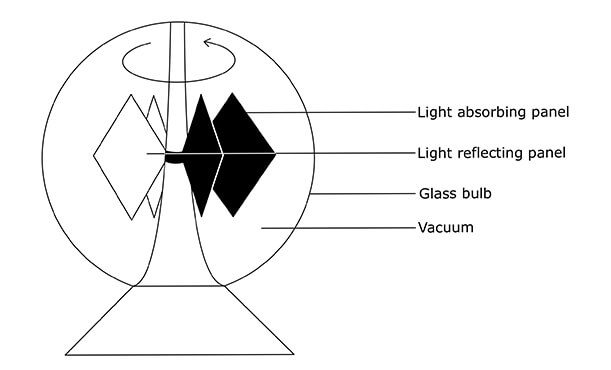
It consists of a glass bulb in which a partial vacuum is present. Inside the bulb, there are four panels.
One side of each panel is white and reflects light. The other side is black and, therefore, absorbs light.
The main chamber of the Crookes solar radiometer is a glass bulb from which air has been removed until a partial vacuum is reached. A rod with four vertically orientated and equally spaced out panels is inside this bulb. One side of each panel is white or reflective, and the other is black. If sunlight, a strong artificial light source, or a heat source reaches the panels, they will begin to turn in the direction of the white sides. Since its creation, many people have tried to explain how this solar radiometer works.
Movement by light
What might seem like a logical explanation for this behaviour is that the movement is caused by momentum from the light particles bouncing off the squares. Crookes even suggested this. However, this theory was disproved soon after the solar radiometer's creation. One way to disprove it was by using a better vacuum inside the bulb. If this theory were correct, it would mean that if fewer molecules are present in the bulb, the squares could move more easily. However, in a stronger vacuum, the squares stayed motionless.
Moreover, if the momentum of the light particles caused the movement, the rod would move in the opposite direction from the observed one. A dark square absorbs some energy from the light and turns it into heat. In contrast, as the name suggests, a reflective surface reflects light. This means the light heats this square less, and more particles bounce off it. So, instead of heat, the particles leave more momentum behind, leading to a push on the white square. Although this pressure on the reflective squares does exist, it is far too small to cause any rotation.
Movement through pressure
A different explanation, still often provided, is that the molecules that encounter the square's warmer side gain some heat, leading to bouncing off at increased speed. These faster-moving molecules would create a larger pressure on the dark side, resulting in the rotation. While it is true that this does lead to a force on the dark side of the square, the faster-moving molecules also make it harder for other molecules to reach that side. Due to the warm molecules acting like a screen for other molecules, this force was not large enough to explain the movement.
Movement through thermal transpiration
Thermal transpiration is currently accepted as the main driving force behind the rotation in the Crookes radiometer. The effect of “thermal creep flow” occurs when the free path length of gas molecules approaches the physical dimension of the macroscopic relevant length scale.
In the presence of a heat source, the black sides of the squares will become warmer than the white sides due to the difference in absorption. Thermal transpiration causes the gas molecules in the surface boundary layers to move to a higher temperature area. This leads to a flow of molecules in front of the white side towards the black side of the square. This, in turn, causes a higher pressure on this side of the square. The larger pressure now acts as a push on the square’s dark side, resulting in the observed movement. Figure 2 schematically shows this phenomenon.
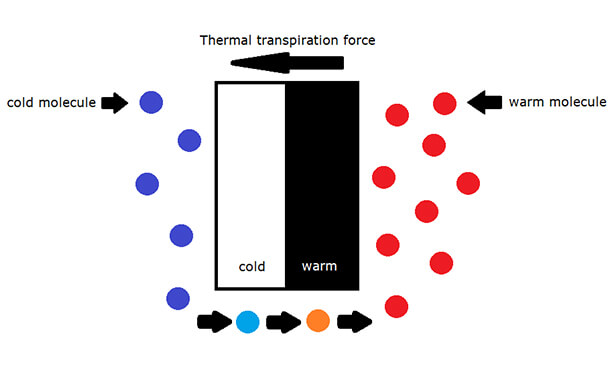
and thermal creep flow work in a near vacuum. The cold molecules on the white side move towards the higher
temperature area on the black side. This leads to a larger pressure on this side, resulting in a pushing force.
So, because the movement is caused by a temperature difference between the white and black sides of the square, any form of sufficient heat can lead to movement. Instead of a light source, the movement can, for example, be started by the heat transferred by laying your hand on the glass. The reverse movement is observed if the glass is rapidly cooled. This is due to the black sides radiating more heat, which cools this side more quickly. So, now the situation is reversed, and the white side is hotter than the black side. The movement stops once the molecules and the squares have equal temperatures.
Due to the need for molecules to create this movement, it is also apparent why a better vacuum does not exhibit the same behaviour. If a lesser, higher-pressure vacuum is present in the chamber, the force caused by the temperature difference is not large enough to sustain movement through the thicker air.
A Crookes radiometer can show the energy captured in sunlight (or any form of electromagnetic radiation), where possibly the speed of rotation is proportional to the radiation energy. However, if we want to accurately and measurably quantify the energy present in sunlight, we must use a different instrument.
Pyranometers
Quantifying solar energy in energy per second passing an imaginary surface area, can be done by using pyranometers. These instruments are more sophisticated than solar radiometers like Crookes radiometers. The operational principle is different in pyranometers, making quantified measurements possible. Obtaining an accurate measurement of solar energy is an indispensable tool in the renewable energy sector, as explained in our article: Solar energy meters: why use them in measuring solar energy. The irradiance is quantified by measuring the power received by the sun per unit of area in Watts per square meter [W/m2]. Two main types of sensors are typically used inside different pyranometers: A thermopile and a silicon photodiode.
Silicon pyranometer
Silicon pyranometers use a silicon photodiode sensor. This low-cost sensor can perform reasonably accurate irradiance measurements with uncertainties in the order of magnitude of +/-10 %. Figure 3 shows a schematic example of a pyranometer with a silicon photodiode.
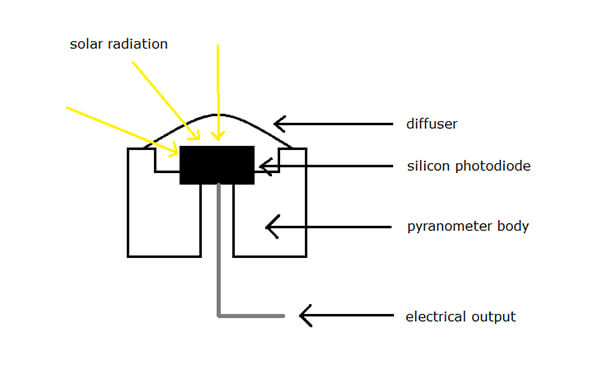
silicon photodiode. This causes an electrical current to flow, which can be measured.
This sensor works using the photoelectric effect, which is the same principle that generates electricity inside solar panels. A description of the photoelectric effect is beyond the scope of this article. But in short, a silicon photodiode experiences a measurable change in its electrical properties when under a light source without using an external power source. This change tells us something about the irradiance that reaches the silicon photodiode.
However, this sensor does have some disadvantages. First, as shown in Figure 4, the value of the response in [W/m2] depends on the wavelength of the light it is exposed to.
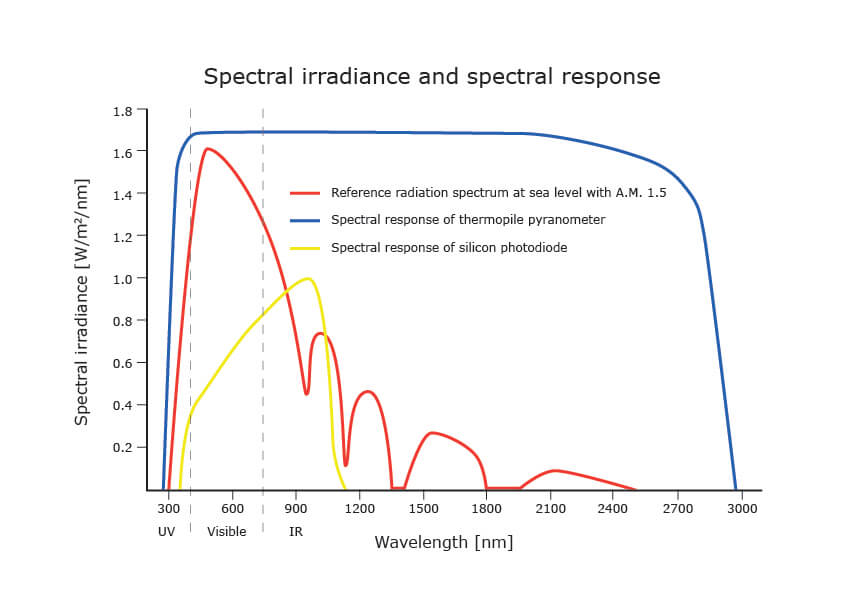
This is undesirable for high-accuracy measurements of W/m2 since it favours some wavelengths of light over others. Moreover, it is not easy to attain the required cosine response. Bare silicon photodiode sensors have trouble measuring irradiance at angles of incidence close to the plane of the silicon surface. As seen in Figure 3, this is partially mitigated by using a diffuser that changes the path of the rays. On top of that, the silicon pyranometer does not capture all wavelengths of the solar radiation that reaches the ground at sea level.
Because of these factors, the accuracy of the performed measurements with silicon pyranometers is limited. This is an issue for larger-scale applications, where strict standards often must be fulfilled. The silicon pyranometers do not meet these standards' requirements, so a different sensor needs to be used.
Thermopile pyranometer
A thermopile can overcome the shortcomings of the silicon pyranometer. Higher-end pyranometers feature these thermopile sensors and provide the highest accuracy irradiance measurements. Figure 5 shows a schematic drawing of a thermopile pyranometer.
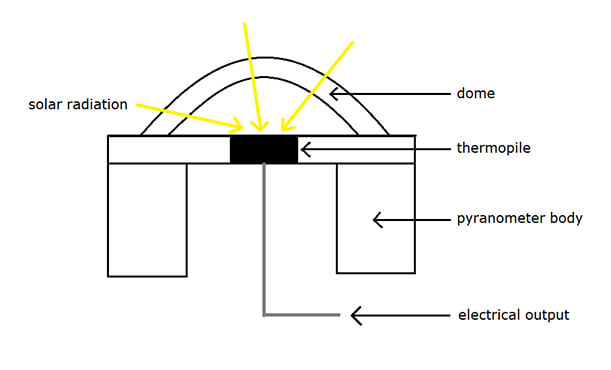
This leads to a temperature difference that causes an electrical voltage to be created, which can be measured.
Thermopiles measure the irradiance by converting a temperature difference (caused by the irradiance) into a small voltage. This, again, is a process that does not need an external power source. In contrast to the spectral response of a silicon photodiode, a black coating on a thermopile equally absorbs every part of the solar spectrum, so it measures the Watts correctly.
Moreover, the response of a thermopile with a properly applied black coating is less mirroring than a photodiode and reacts with an ideal cosine response on the angle of incoming sunlight. Higher accuracy is also guaranteed through lower temperature dependence and a more stable response over time. For more detailed information on thermopiles, see our article: What is a thermopile?
Due to these advantages over the silicon pyranometer, the thermopile sensor satisfies the standards set for the highest accuracy solar irradiation measurements, capable of attaining uncertainties in the order of ± 2%. We at Hukseflux offer various thermopile pyranometers to meet different requirements.
Conclusion and comparison table
One hundred fifty years ago, Sir William Crookes invented the first solar radiometer, which showcases the energy captured in light. How this works has been widely discussed since its creation, but the most accepted explanation is that thermal transpiration is the main driving force. However, if we wish to quantify solar energy accurately, we need to use pyranometers. Pyranometers are based either on a silicon photodiode or a thermopile sensor. The latter offers the highest accuracy measurements, meeting requirements of the strictest standards. The following table provides a summary of the comparison of the three sensors.
DOES IT QUANTIFY THE SOLAR IRRADIANCE? | APPLICATION | STANDARDISED | |
Crookes solar radiometer | No | Gadget at home or in the classroom | No |
Silicon pyranometer | Yes [W/m2], but not the whole spectrum Accuracies in the order of ± 10% | Agriculture, analysis of PV systems where high-accuracy measurements are not needed
| No |
Thermopile pyranometer | Yes (W/m2) Accuracies up to ± 2% | Professional analysis of solar energy and PV systems and high-accuracy meteorology. | Yes, ISO 9060 |
If this article has left you interested in further knowledge on pyranometers, be sure to read the other articles in our Library or get in contact with our skilled team.