Pyranometers: all you need to know
A pyranometer measures solar irradiance on flat surfaces, capturing radiation in W/m² from a hemispherical view (180°). A pyranometer or solar irradiance sensor is used for climatological research and weather monitoring. However, in recent years, the use of pyranometers in the solar energy sector has increased. This article will explain more about what it measures, what it is used for, how it works, and the types of pyranometer sensors.
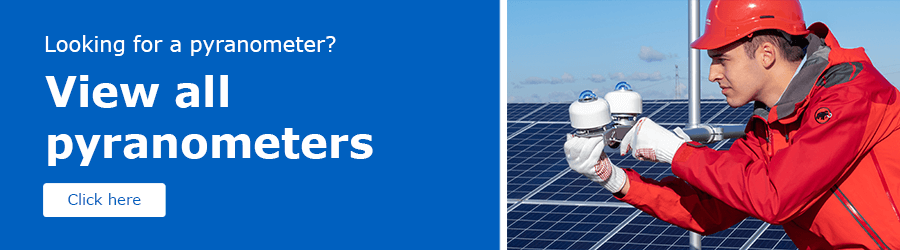
What does a pyranometer measure?
A pyranometer measures the global irradiance in watts per square meter (W/m2). The global irradiance is the amount of solar energy per unit area per unit of time, which comes from direct and diffuse solar radiation. Direct radiation, or direct sunlight, travels straight from the sun to the earth without interruption by clouds or atmospheric particles. Diffuse radiation is the sunlight that is scattered by water droplets, air molecules and reflected from buildings, trees, etc. Measuring both components is crucial for understanding the solar energy availability at a particular site. The pyranometer sensor converts this global irradiance into an electrical signal that can be measured.
What are pyranometers used for?
The pyranometer sensor’s main role is solar monitoring. Solar monitoring measures the intensity, duration and availability of sunlight. Measuring these factors is most commonly used in the following sectors:
- Solar energy sector
Of course, pyranometers are indispensable in the solar energy sector. They are essential to assess the performance of solar panels and photovoltaic (PV) power plants. Through solar monitoring, you can optimise the positioning of your solar installation and calculate the potential energy yield.
- Agriculture
A pyranometer can also be helpful if you want to optimise crop growth and irrigation systems. If you know when and how much solar energy is available, you can make better decisions on planting schedules, crop types, and irrigation needs. In greenhouses, the data from pyranometers help control the light levels to ensure optimal plant growth.
- Meteorology and climate science
Measuring solar irradiance is also useful for studying weather patterns and atmospheric conditions. The data from a pyranometer sensor is pivotal in determining long-term weather forecasts and climate models.
- Building and urban planning
It might be useful for an architect to understand solar radiation before building and urban planning. So they can create designs that leverage sunlight effectively. Especially in recent years, sustainable urban development has become more important. A pyranometer will improve buildings with efficient energy consumption and maximise sunlight availability.
In each of these applications, the accuracy and reliability of a pyranometer sensor is most important. Hukseflux is a leading provider of various pyranometers and solar radiation sensors. Please check our solar radiation sensors for more information.
How do pyranometers work?
There are two main types of pyranometers: a thermopile and a silicon photodiode pyranometer. Here, we will discuss how a thermopile pyranometer works since Hukseflux uses this technology. Hukseflux uses thermopile pyranometers instead of silicon photodiodes because they have a
- wider spectral range: measuring irradiance from 0.3 to 2.8 micrometres (difference shown in Figure 1)
- directional sensitivity: less sensitive to the angle of incoming sunlight
- more stable response over time
- less temperature dependency
- high accuracy
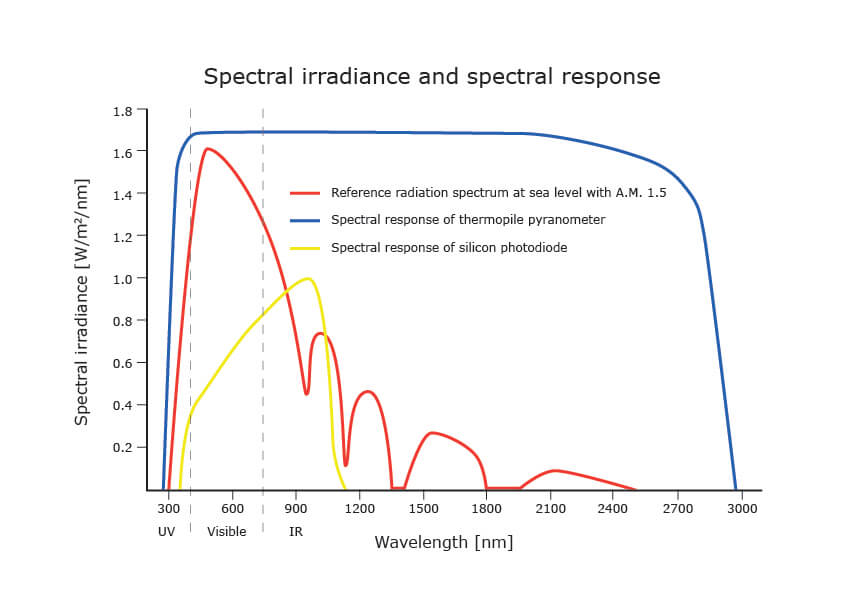
When measuring irradiance, it is required that the “beam radiation” (direct sunlight) varies according to the cosine of the angle of incidence. For direct sunlight, the angle of incidence is 0°. Ideally, the pyranometer should have a full response to this direct sunlight. If the sun is on the horizon, the angle of incidence is near 90°. When this is the case, the pyranometer should also have a near-zero response. For other angles, the response should decrease proportionally. The formula for the ideal response R at a given angle θ is:
A pyranometer must vary according to the cosine rule because it represents the true power of the sunlight as it strikes the surface.
Classes of pyranometers
At Hukseflux, our pyranometers usually consist of a thermopile sensor with a black coating, one or two glass domes, an anodised aluminium body, and a housing. Hukseflux has various pyranometers to satisfy every customer’s needs.
The sensors can be classified through certain specification parameters. ISO (the International Organization for Standardization) is a worldwide federation of national standards bodies. For measuring solar radiation in pyranometers (and pyrheliometers), ISO 9060 is used. ISO collaborates closely with the IEC (International Electrotechnical Commission) (IEC), which prepares and publishes international standards for all electrical, electronic, and related technologies.
In 2018, a new version of ISO 9060 was published. If you compare it to the previous standard from 1990, the latest version includes temperature dependence and directional response. Overall, the newer version has stricter requirements than the prior 1990 version.
In Table 1, you can see the new and old class names, the specification parameters, and the acceptance intervals. There are three instrument accuracy classes: A, B, and C, with class A being the most accurate. Each of these classes includes a special extension called “spectrally flat”. If we say a pyranometer is spectrally flat, we mean that it measures solar radiation in a way that does not favour or neglect any part of the sunlight’s spectrum. Furthermore, Table 1 gives an example of a Hukseflux pyranometer that classifies for these new standards.
NOTE: Table 1 briefly overviews the specifications and their values. The original pyranometer classification list includes more information on the specification parameters and has guard bands (intervals between a tolerance limit).
Table 1: Pyranometer classification list
Specification parameter | Name of the classes and acceptance intervals | ||
Name of the class ISO 9060:2018 | A | B | C |
Roughly corresponding |
Secondary standard |
First class |
Second class |
Response time | < 10 s | < 20 s | < 30 s |
Zero off-set A Zero off-set B Zero off-set C | ± 7 W/m2 ± 2 W/m2 ± 10 W/m2 | ± 15 W/m2 ± 4 W/m2 ± 21 W/m2 | ± 30 W/m2 ± 8 W/m2 ± 41 W/m2 |
Non-stability | ± 0.8 % | ± 1.5 % | ± 3 % |
Nonlinearity | ± 0.5 % | ± 1 % | ± 3 % |
Directional response | ± 10 W/m2 | ± 20 W/m2 | ± 30 W/m2 |
Spectral error | ± 0.5 % | ± 1 % | ± 5 % |
Temperature response | ± 1 % | ± 2 % | ± 4 % |
Tilt-response | ± 0.5 % | ± 2 % | ± 5 % |
Additional signal processing errors | ± 2 W/m2 | ± 5 W/m2 | ± 10 W/m2 |
Hukseflux pyranometers that classify for these classes | SR30-M2-D1 SR25 SR22 SR20-D2 SR20 | SR15-D1 SR15-D2A2 SR15-A1
| SR05-D1A3 SR05-D2A2 SR05-A1 |
![]() | ![]()
| ![]() |
A short explanation of the specification parameters:
- Response time: a measure of the stabilisation period for an accurate reading under realistic irradiance changes. Time for 95% response.
- Zero off-set: a measure of the stability of the zero-point specified for the effect of thermal radiation, temperature transient, and other influences. Zero off-set A: response to -200 W/m2 net thermal radiation. Zero off-set B: response to 5 K/h change in ambient temperature. Zero-off-set C: total zero-off-set including A and B and other sources.
- Non-stability: a measure of the long-term stability, assuming regular and proper maintenance, including cleaning of the pyranometer. Percentage change in responsivity per year.
- Nonlinearity: the level of irradiance. Percentage deviation from the responsivity at 500 W/m² due to the change in irradiance within 100 W/m² to 1000 W/m².
- Directional response: a measure of the deviations from the ideal “cosine behaviour” and its azimuthal variation. The range of errors caused by assuming that the normal incidence responsivity is valid for all directions when measured from any direction (with an incidence angle of up to 90° or even from below the sensor) a beam radiation whose normal incidence irradiance is 1000 W/m².
- Spectral error: a measure of the deviation of the spectral responsivity from a completely flat spectral responsivity.
- Temperature response: temperature of the radiometer body. Percentage deviation due to the change in ambient temperature within the interval from -10° C to 40° C relative to the signal at 20° C.
- Tilt-response: tilt angle of the receiving surface. Percentage deviation from the responsivity at 0° tilt (horizontal) due to change in tilt from 0° to 180° at 1000 W/m² irradiance.
Conclusion
A pyranometer measures solar radiation in W/m² from a hemispherical view. With the use of thermopiles, the incoming solar radiation can be measured. Solar energy is everywhere, and knowing how much is available at a certain sight is crucial. Whether for setting up solar panels, growing crops, or predicting the weather. As more people consider green energy and sustainable improvements, solar radiation sensors and, thus, pyranometers become even more important.
Hukseflux provides pyranometers for each class. If you want to order a pyranometer, ask yourself how much accuracy you need. Class A pyranometers have the highest accuracy, followed by Class B and Class C. More accuracy comes with higher prices, more maintenance, and a deeper understanding of the device. Our skilled team is here to help you if you need help determining which solar radiation sensor you need. Just get in touch with us through our contact page.