Pyranometer physics: why two domes?
Pyranometers are accurate instruments to measure solar irradiance. Have you ever wondered why thermopile pyranometers have one or two domes? This note explores the physics of pyranometers: how do thermopile pyranometers work and why are these domes necessary?
Read the full article here: Pyranometer physics: why two domes? - note (PDF)
Introduction
All thermopile pyranometers are thermal instruments: they derive their signal from a temperature difference in the sensor. In other words: thermopile pyranometers are just fancy thermometers. The trick is to make sure the pyranometer is only sensitive to solar radiation.
This note attempts to give you an understanding of the pyranometer working principle and, in particular, an answer to the question: why do accurate pyranometers use two glass domes?
Pyranometer basics
As the name implies, the central element in any thermopile pyranometer is the thermopile. A thermopile is a stack of two different conducting materials. It generates a voltage in response to a temperature difference between the ‘hot’ and the ‘cold’ side of the stack. This phenomenon is known as the ‘thermoelectric effect’, expressed by the different Seebeck coefficients of the two materials.
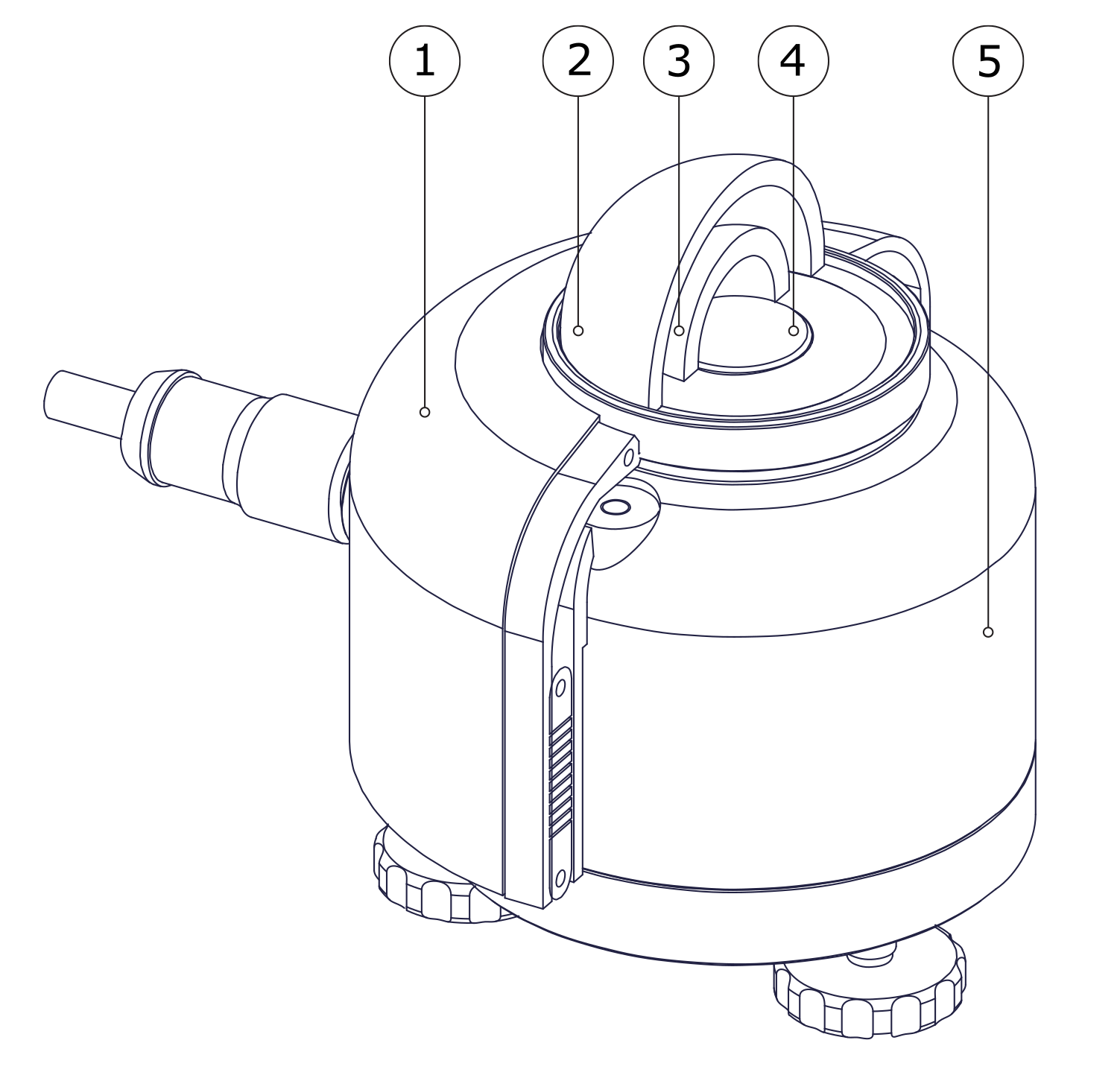
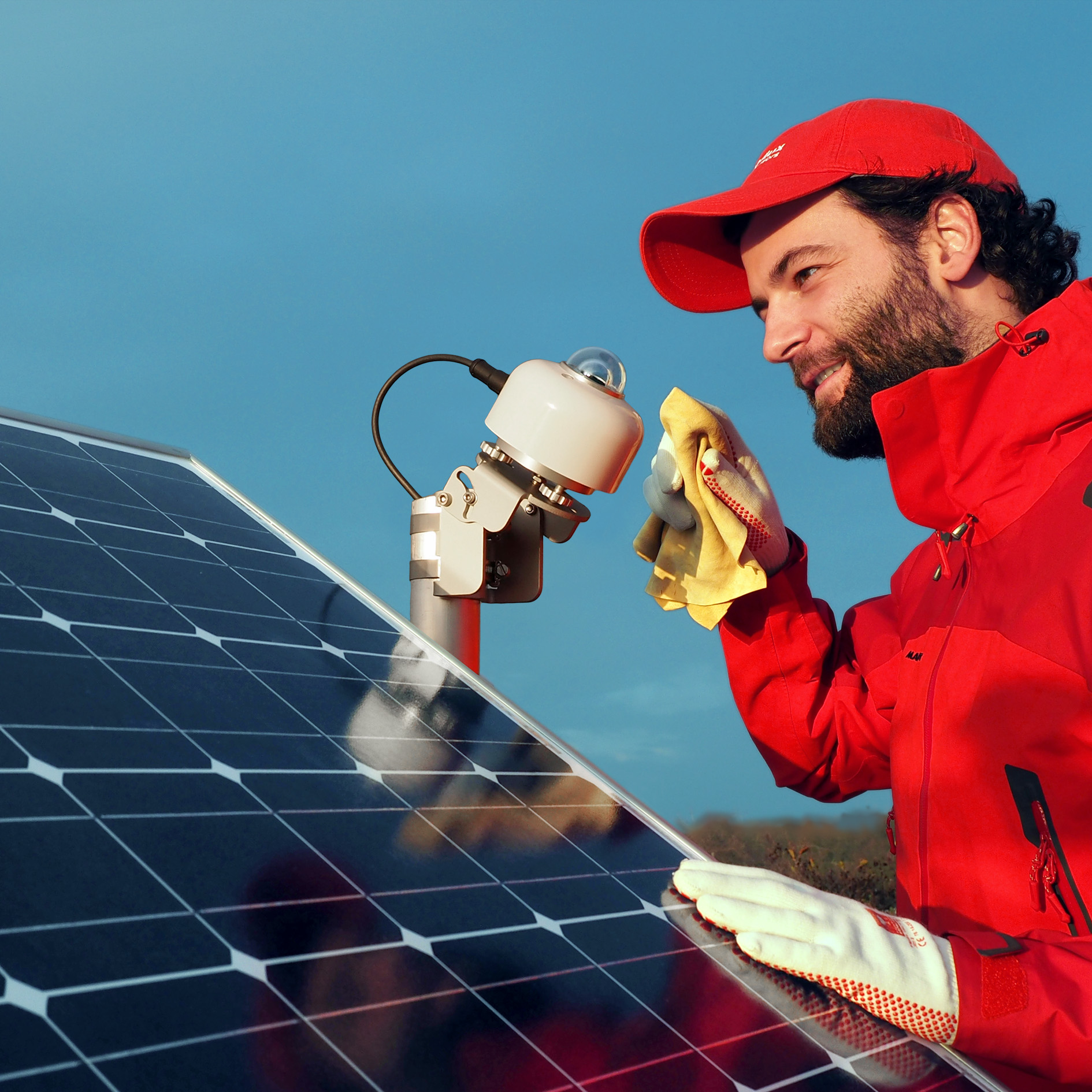
The cold side of the thermopile is attached to the sensor body, while the hot side, the ‘sensor surface’, is coated with a black, radiation-absorbing coating and exposed to the sun. Solar radiation will heat up the sensor surface, while the cold side is kept at ambient temperature via the thermal connection to the sensor body. This temperature difference is what generates the sensor signal.
The rest of the pyranometer is designed to ensure that all other thermal exchange is reduced as much as possible. Only solar radiation should be allowed to heat up the sensor surface. In this note, we will focus on one unwanted thermal exchange process: the exchange of infrared radiation with the cold sky.
Pyranometer performance is standardized in ISO 9060[1], based on several criteria. The offset signal caused by infrared exchange is one of these criteria, known as ‘zero offset a’. For accurate measurements, it is important to reduce this offset as much as possible.
This note will provide insight in how we achieve a low ‘zero offset a’ in the Hukseflux range of next-level pyranometers: SR05, SR15 and SR30.
Introducing the first dome
A typical black coating used in pyranometer construction has a flat spectral sensitivity in the range of 200 nm (ultraviolet) to 50 μm (far infrared). Therefore, a bare thermopile pyranometer would be very sensitive to non-solar infrared radiation. In particular, the sensor surface, which has a temperature close to the ambient air temperature, would exchange infrared radiation with the cold sky above. A bare thermopile would also be sensitive to convective cooling by wind, as well as rain, snow etc.
On a clear, cloudless day, the atmosphere can be estimated by a black body with a temperature of -20 °C, meaning that the infrared exchange between the sensor and sky will actually cool down the sensor surface. In response, the sensor will underestimate the solar irradiance. We call this the ‘longwave sensitivity’ of the sensor, analogous to the desired shortwave sensitivity for solar radiation.
This effect is especially visible on clear, cloudless nights where pyranometers typically report a negative sensor signal: the so-called ‘zero offset a’. Note that this offset is always present: during the day it is buried beneath or hidden within the positive signal generated by solar irradiance. The black body temperature of a clouded sky is much closer to the air temperature at ground level, such that this effect is much smaller on clouded days and nights.
A single glass dome, as mounted on Class C pyranometers like SR05, is the first line of defence against convection as well as this offset. Glass transmits radiation with a near-flat spectral sensitivity in the 285-3000 nm range, while absorbing radiation in most of the infrared range. The dome will then act as a radiation shield for infrared radiation: thermal exchange will now take place between sky and dome and between dome and sensor surface separately. Box 1 describes these physics in more detail.
It also acts as a perfect protection against convection and precipitation. The dome-shape gives the sensor 180˚ field of view, which is a requirement for a pyranometer. Thermal connection of the sensor body and the dome leads to thermal equilibrium between dome and sensor, reducing thermal exchange even further.
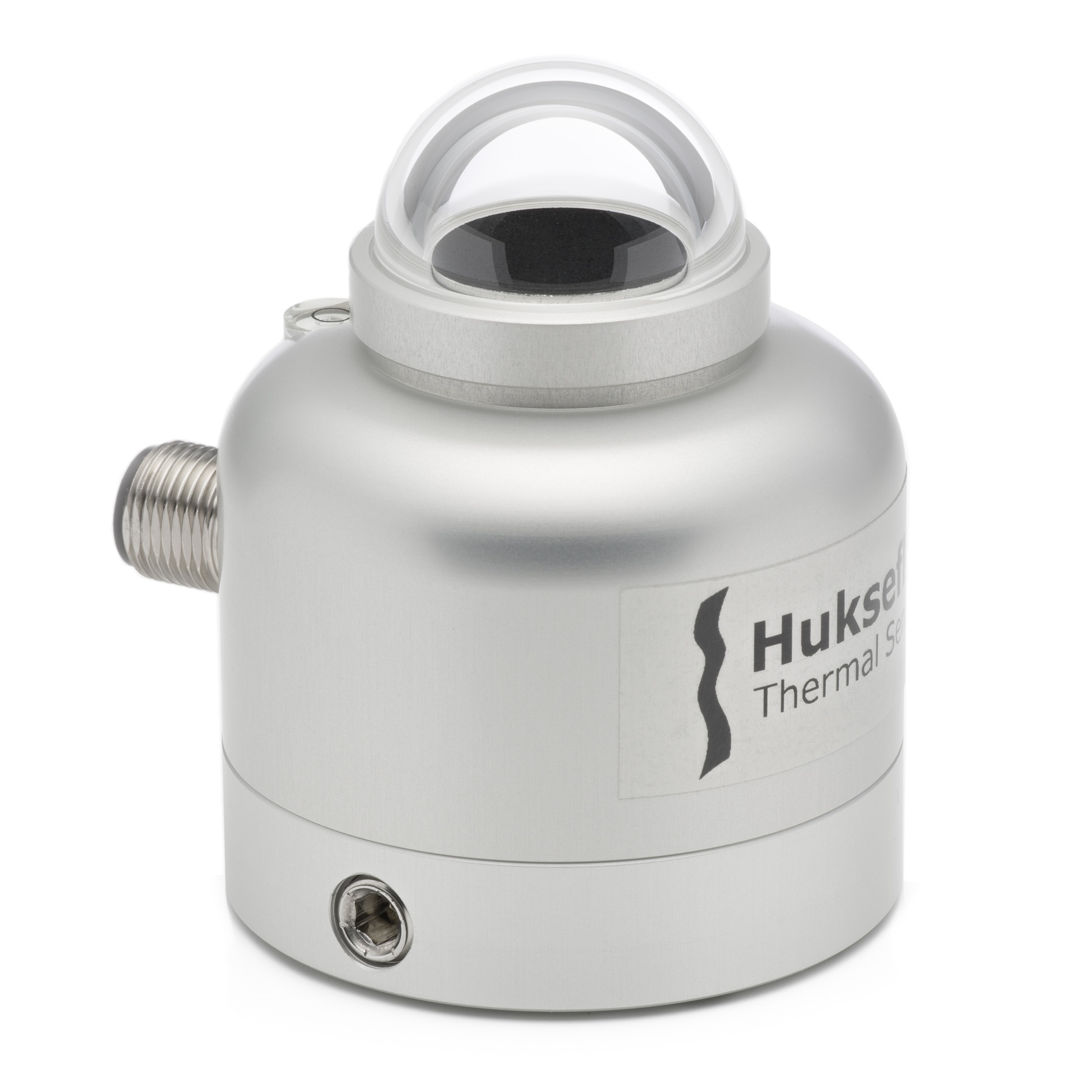
More is better?
As shown in Box 1, the insulation against infrared thermal exchange increases with the number of domes. That’s why our Class A and B pyranometers like SR30 and SR15 are equipped with two domes instead of one.
However, every added dome yields a lower increase in infrared insulation than the previous one: an example of the law of diminishing returns. Since domes are expensive, it is not effective to use more than two domes. Moreover, other problems like reduced transmission and increased lens effect complicate the construction of an accurate pyranometer with more than two domes.
Further improvements
Hukseflux’ SR30 spectrally flat Class C (secondary standard0 pyranometers employ an additional technique to reduce infrared measurement offsets even further: Recirculating Ventilation and Heating (RVH).
A ventilator recirculates air through the sensor body and between the inner and outer domes to create a uniform temperature distribution throughout the sensor. Ideally, the sensor surface and inner dome will be at the same temperature, and a net exchange of zero is measured. Results indeed show that the longwave sensitivity of the sensor is very low.
In addition, SR30 is equipped with a heater to prevent rime, dew and frost on cold days. The recirculated air also makes sure that the heating power is spread uniformly throughout the sensor, reducing thermal gradients that would otherwise provide a measurement offset.
...
Read the full article here: